图书介绍
Molecular cell biology sixth edition【2025|PDF|Epub|mobi|kindle电子书版本百度云盘下载】
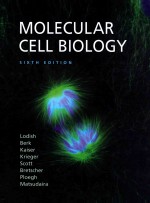
- harvey ldish and arnold berk and chris a.kaiser and monty krieger and matthew p.scott 著
- 出版社: w.h.freeman and company
- ISBN:1429203142
- 出版时间:2008
- 标注页数:1229页
- 文件大小:387MB
- 文件页数:1269页
- 主题词:
PDF下载
下载说明
Molecular cell biology sixth editionPDF格式电子书版下载
下载的文件为RAR压缩包。需要使用解压软件进行解压得到PDF格式图书。建议使用BT下载工具Free Download Manager进行下载,简称FDM(免费,没有广告,支持多平台)。本站资源全部打包为BT种子。所以需要使用专业的BT下载软件进行下载。如BitComet qBittorrent uTorrent等BT下载工具。迅雷目前由于本站不是热门资源。不推荐使用!后期资源热门了。安装了迅雷也可以迅雷进行下载!
(文件页数 要大于 标注页数,上中下等多册电子书除外)
注意:本站所有压缩包均有解压码: 点击下载压缩包解压工具
图书目录
Part Ⅰ Chemical and Molecular Foundations1
1 LIFE BEGINS WITH CELLS1
1.1 The Diversity and Commonality of Cells1
All Cells Are Prokaryotic or Eukaryotic1
Unicellular Organisms Help and Hurt Us4
Viruses Are the Ultimate Parasites6
Changes in Cells Underlie Evolution6
Even Single Cells Can Have Sex7
We Develop from a Single Cell8
Stem Cells,Fundamental to Forming Tissues and Organs,Offer Medical Opportunities8
1.2 The Molecules of a Cell9
Small Molecules Carry Energy,Transmit Signals,and Are Linked into Macromolecules9
Proteins Give Cells Structure and Perform Most Cellular Tasks10
Nucleic Acids Carry Coded Information for Making Proteins at the Right Time and Place11
The Genome Is Packaged into Chromosomes and Replicated During Cell Division12
Mutations May Be Good,Bad,or Indifferent13
1.3 The Work of Cells14
Cells Build and Degrade Numerous Molecules and Structures15
Animal Cells Produce Their Own External Environment and Glues16
Cells Change Shape and Move16
Cells Sense and Send Information16
Cells Regulate Their Gene Expression to Meet Changing Needs17
Cells Grow and Divide18
Cells Die from Aggravated Assault or an Internal Program19
1.4 Investigating Cells and Their Parts20
Cell Biology Reveals the Size,Shape,Location,and Movements of Cell Components20
Biochemistry and Biophysics Reveal the Molecular Structure and Chemistry of Purified Cell Constituents21
Genetics Reveals the Consequences of Damaged Genes22
Genomics Reveals Differences in the Structure and Expression of Entire Genomes23
Developmental Biology Reveals Changes in the Properties of Cells as They Specialize23
Choosing the Right Experimental Organism for the Job25
The Most Successful Biological Studies Use Multiple Approaches27
1.5 A Genome Perspective on Evolution28
Metabolic Proteins,the Genetic Code,and Organelle Structures Are Nearly Universal28
Darwin’s Ideas About the Evolution of Whole Animals Are Relevant to Genes28
Many Genes Controlling Development Are Remarkably Similar in Humans and Other Animals28
Human Medicine Is Informed by Research on Other Organisms29
2 CHEMICAL FOUNDATIONS31
2.1 Covalent Bonds and Noncovalent Interactions32
The Electronic Structure of an Atom Determines the Number and Geometry of Covalent Bonds It Can Make33
Electrons May Be Shared Equally or Unequally in Covalent Bonds34
Covalent Bonds Are Much Stronger and More Stable Than Noncovalent Interactions35
Ionic Interactions Are Attractions between Oppositely Charged Ions36
Hydrogen Bonds Determine the Water Solubility of Uncharged Molecules37
Van der Waals Interactions Are Caused by Transient Dipoles37
The Hydrophobic Effect Causes Nonpolar Molecules to Adhere to One Another38
Molecular Complementarity Mediated via Noncovalent Interactions Permits Tight,Highly Specific Binding of Biomolecules39
2.2 Chemical Building Blocks of Cells40
Amino Acids Differing Only in Their Side Chains Compose Proteins41
Five Different Nucleotides Are Used to Build Nucleic Acids44
Monosaccharides Joined by Glycosidic Bonds Form Linear and Branched Polysaccharides44
Phospholipids Associate Noncovalently to Form the Basic Bilayer Structure of Biomembranes46
2.3 Chemical Equilibrium49
Equilibrium Constants Reflect the Extent of a Chemical Reaction50
Chemical Reactions in Cells Are at Steady State50
Dissociation Constants of Binding Reactions Reflect the Affinity of Interacting Molecules50
Biological Fluids Have Characteristic pH Values51
Hydrogen Ions Are Released by Acids and Taken Up by Bases52
Buffers Maintain the pH of Intracellular and Extracellular Fluids52
2.4 Biochemical Energetics54
Several Forms of Energy Are Important in Biological Systems54
Cells Can Transform One Type of Energy into Another55
The Change in Free Energy Determines the Direction of a Chemical Reaction55
The △G°’ of a Reaction Can Be Calculated from Its Keq56
The Rate of a Reaction Depends on the Activation Energy Necessary to Energize the Reactants into a Transition State56
Life Depends on the Coupling of Unfavorable Chemical Reactions with Energetically Favorable Reactions57
Hydrolysis of ATP Releases Substantial Free Energy and Drives Many Cellular Processes57
ATP Is Generated During Photosynthesis and Respiration59
NAD+ and FAD Couple Many Biological Oxidation and Reduction Reactions59
3 PROTEIN STRUCTURE AND FUNCTION63
3.1 Hierarchical Structure of Proteins64
The Primary Structure of a Protein Is Its Linear Arrangement of Amino Acids65
Secondary Structures Are the Core Elements of Protein Architecture66
Overall Folding of a Polypeptide Chain Yields Its Tertiary Structure67
Different Ways of Depicting the Conformation of Proteins Convey Different Types of Information68
Structural Motifs Are Regular Combinations of Secondary and Tertiary Structures68
Structural and Functional Domains Are Modules of Tertiary Structure70
Proteins Associate into Multimeric Structures and Macromolecular Assemblies72
Members of Protein Families Have a Common Evolutionary Ancestor72
3.2 Protein Folding74
Planar Peptide Bonds Limit the Shapes into Which Proteins Can Fold74
Information Directing a Protein’s Folding Is Encoded in Its Amino Acid Sequence74
Folding of Proteins in Vivo Is Promoted by Chaperones75
Alternatively Folded Proteins Are Implicated in Diseases77
3.3 Protein Function78
Specific Binding of Ligands Underlies the Functions of Most Proteins78
Enzymes Are Highly Efficient and Specific Catalysts79
An Enzyme’s Active Site Binds Substrates and Carries Out Catalysis80
Serine Proteases Demonstrate How an Enzyme’s Active Site Works81
Enzymes in a Common Pathway Are Often Physically Associated with One Another84
Enzymes Called Molecular Motors Convert Energyinto Motion85
3.4 Regulating Protein Function Ⅰ:Protein Degradation86
Regulated Synthesis and Degradation of Proteins is a Fundamental Property of Cells86
The Proteasome Is a Complex Molecular Machine Used to Degrade Proteins87
Ubiquitin Marks Cytosolic Proteins for Degradation in Proteasomes88
3.5 Regulating Protein Function Ⅱ:Noncovalent and Covalent Modifications88
Noncovalent Binding Permits Allosteric,or Cooperative,Regulation of Proteins89
Noncovalent Binding of Calcium and GTP Are Widely Used As Allosteric Switches to Control Protein Activity90
Phosphorylation and Dephosphorylation Covalently Regulate Protein Activity91
Proteolytic Cleavage Irreversibly Activates or Inactivates Some Proteins91
Higher-Order Regulation Includes Control of Protein Location and Concentration92
3.6 Purifying,Detecting,and Characterizing Proteins92
Centrifugation Can Separate Particles and Molecules That Differ in Mass or Density92
Electrophoresis Separates Molecules on the Basis of Their Charge-to-Mass Ratio94
Liquid Chromatography Resolves Proteins by Mass,Charge,or Binding Affinity96
Highly Specific Enzyme and Antibody Assays Can Detect Individual Proteins98
Radioisotopes Are Indispensable Tools for Detecting Biological Molecules99
Mass Spectrometry Can Determine the Mass and Sequence of Proteins101
Protein Primary Structure Can Be Determined by Chemical Methods and from Gene Sequences103
Protein Conformation Is Determined by Sophisticated Physical Methods103
3.7 Proteomics105
Proteomics Is the Study of All or a Large Subset of Proteins in a Biological System105
Advanced Techniques in Mass Spectrometry Are Critical to Proteomic Analysis106
Part Ⅱ Genetics and Molecular Biology111
4 BASIC MOLECULAR GENETIC MECHANISMS111
4.1 Structure of Nucleic Acids113
A Nucleic Acid Strand Is a Linear Polymer with End-to-End Directionality113
Native DNA Is a Double Helix of Complementary Antiparallel Strands114
DNA Can Undergo Reversible Strand Separation116
Torsional Stress in DNA Is Relieved by Enzymes117
Different Types of RNA Exhibit Various Conformations Related to Their Functions118
4.2 Transcription of Protein-Coding Genes and Formation of Functional mRNA120
A Template DNA Strand Is Transcribed into a Complementary RNA Chain by RNA Polymerase120
Organization of Genes Differs in Prokaryotic and Eukaryotic DNA122
Eukaryotic Precursor mRNAs Are Processed to Form Functional mRNAs123
Alternative RNA Splicing Increases the Number of Proteins Expressed from a Single Eukaryotic Gene125
4.3 The Decoding of mRNA by tRNAs127
Messenger RNA Carries information from DNA in a Three-Letter Genetic Code127
The Folded Structure of tRNA Promotes Its Decoding Functions129
Nonstandard Base Pairing Often Occurs Between Codons and Anticodons130
Amino Acids Become Activated When Covalently Linked to tRNAs131
4.4 Stepwise Synthesis of Proteins on Ribosomes132
Ribosomes Are Protein-Synthesizing Machines132
Methionyl-tRNAiMET Recognizes the AUG Start Codon133
Translation Initiation Usually Occurs at the First AUG from the 5’ End of an mRNA133
During Chain Elongation Each Incoming Aminoacyl-tRNA Moves Through Three Ribosomal Sites135
Translation Is Terminated by Release Factors When a Stop Codon Is Reached137
Polysomes and Rapid Ribosome Recycling Increase the Efficiency of Translation138
4.5 DNA Replication139
DNA Polymerases Require a Primer to Initiate Replication140
Duplex DNA Is Unwound and Daughter Strands Are Formed at the DNA Replication Fork141
Several Proteins Participate in DNA Replication141
DNA Replication Usually Occurs Bidirectionally from Each Origin143
4.6 DNA Repair and Recombination145
DNA Polymerases Introduce Copying Errors and Also Correct Them145
Chemical and Radiation Damage to DNA Can Lead to Mutations145
High-Fidelity DNA Excision-Repair Systems Recognize and Repair Damage147
Base Excision Repairs T·G Mismatches and Damaged Bases147
Mismatch Excision Repairs Other Mismatches and Small Insertions and Deletions147
Nucleotide Excision Repairs Chemical Adducts That Distort Normal DNA Shape148
Two Systems Utilize Recombination to Repair Double-Strand Breaks in DNA149
Homologous Recombination Can Repair DNA Damage and Generate Genetic Diversity150
4.7 Viruses:Parasites of the Cellular Genetic System154
Most Viral Host Ranges Are Narrow154
Viral Capsids Are Regular Arrays of One or a Few Types of Protein154
Viruses Can Be Cloned and Counted in Plaque Assays155
Lytic Viral Growth Cycles Lead to the Death of Host Cells156
Viral DNA Is Integrated into the Host-Cell Genome in Some Nonlytic Viral Growth Cycles158
5 MOLECULAR GENETIC TECHNIQUES165
5.1 Genetic Analysis of Mutations to Identify and Study Genes166
Recessive and Dominant Mutant Alleles Generally Have Opposite Effects on Gene Function166
Segregation of Mutations in Breeding Experiments Reveals Their Dominance or Recessivity167
Conditional Mutations Can Be Used to Study Essential Genes in Yeast170
Recessive Lethal Mutations in Diploids Can Be Identified by Inbreeding and Maintained in Heterozygotes171
Complementation Tests Determine Whether Different Recessive Mutations Are in the Same Gene171
Double Mutants Are Useful in Assessing the Order in Which Proteins Function171
Genetic Suppression and Synthetic Lethality Can Reveal Interacting or Redundant Proteins173
Genes Can Be Identified by Their Map Position on the Chromosome174
5.2 DNA Cloning and Characterization176
Restriction Enzymes and DNA Ligases Allow Insertion of DNA Fragments into Cloning Vectors176
E.coli Plasmid Vectors Are Suitable for Cloning Isolated DNA Fragments178
cDNA Libraries Represent the Sequences of Protein-Coding Genes179
cDNAs Prepared by Reverse Transcription of Cellular mRNAs Can Be Cloned to Generate cDNA Libraries181
DNA Libraries Can Be Screened by Hybridization to an Oligonucleotide Probe181
Yeast Genomic Libraries Can Be Constructed with Shuttle Vectors and Screened by Functional Complementation182
Gel Electrophoresis Allows Separation of Vector DNA from Cloned Fragments184
Cloned DNA Molecules Are Sequenced Rapidly by the Dideoxy Chain-Termination Method187
The Polymerase Chain Reaction Amplifies a Specific DNA Sequence from a Complex Mixture188
5.3 Using Cloned DNA Fragments to Study Gene Expression191
Hybridization Techniques Permit Detection of Specific DNA Fragments and mRNAs191
DNA Microarrays Can Be Used to Evaluate the Expression of Many Genes at One Time192
Cluster Analysis of Multiple Expression Experiments Identifies Co-regulated Genes193
E.coli Expression Systems Can Produce Large Quantities of Proteins from Cloned Genes194
Plasmid Expression Vectors Can Be Designed for Use in Animal Cells196
5.4 Identifying and Locating Human Disease Genes198
Many Inherited Diseases Show One of Three Major Patterns of Inheritance199
DNA Polymorphisms Are Used in Linkage-Mapping Human Mutations200
Linkage Studies Can Map Disease Genes with a Resolution of About 1 Centimorgan201
Further Analysis Is Needed to Locate a Disease Gene in Cloned DNA202
Many Inherited Diseases Result from Multiple Genetic Defects203
5.5 Inactivating the Function of Specific Genes in Eukaryotes204
Normal Yeast Genes Can Be Replaced with Mutant Alleles by Homologous Recombination205
Transcription of Genes Ligated to a Regulated Promoter Can Be Controlled Experimentally206
Specific Genes Can Be Permanently Inactivated in the Germ Line of Mice207
Somatic Cell Recombination Can Inactivate Genes in Specific Tissues208
Dominant-Negative Alleles Can Functionally Inhibit Some Genes209
RNA Interference Causes Gene Inactivation by Destroying the Corresponding mRNA210
6 GENES,GENOMICS,AND CHROMOSOMES215
6.1 Eukaryotic Gene Structure217
Most Eukaryotic Genes Contain Introns and Produce mRNAs Encoding Single Proteins217
Simple and Complex Transcription Units Are Found in Eukaryotic Genomes217
Protein-Coding Genes May Be Solitary or Belong to a Gene Family219
Heavily Used Gene Products Are Encoded by Multiple Copies of Genes221
Nonprotein-Coding Genes Encode Functional RNAs222
6.2 Chromosomal Organization of Genes and Noncoding DNA223
Genomes of Many Organisms Contain Much Nonfunctional DNA223
Most Simple-Sequence DNAs Are Concentrated in Specific Chromosomal Locations224
DNA Fingerprinting Depends on Differences in Length of Simple-Sequence DNAs225
Unclassified Spacer DNA Occupies a Significant Portion of the Genome225
6.3 Transposable(Mobile) DNA Elements226
Movement of Mobile Elements Involves a DNA or an RNA Intermediate226
DNA Transposons Are Present in Prokaryotes and Eukaryotes227
LTR Retrotransposons Behave Like Intracellular Retroviruses229
Non-LTR Retrotransposons Transpose by a Distinct Mechanism230
Other Retrotransposed RNAs Are Found in Genomic DNA234
Mobile DNA Elements Have Significantly Influenced Evolution234
6.4 Organelle DNAs236
Mitochondria Contain Multiple mtDNA Molecules237
mtDNA Is Inherited Cytoplasmically237
The Size,Structure,and Coding Capacity of mtDNA Vary Considerably Between Organisms238
Products of Mitochondrial Genes Are Not Exported240
Mitochondria Evolved from a Single Endosymbiotic Event Involving a Rickettsia-like Bacterium240
Mitochondrial Genetic Codes Differ from the Standard Nuclear Code240
Mutations in Mitochondrial DNA Cause Several Genetic Diseases in Humans240
Chloroplasts Contain Large DNAs Often Encoding More Than a Hundred Proteins242
6.5 Genomics:Genome-wide Analysis of Gene Structure and Expression243
Stored Sequences Suggest Functions of Newly Identified Genes and Proteins243
Comparison of Related Sequences from Different Species Can Give Clues to Evolutionary Relationships Among Proteins244
Genes Can Be Identified Within Genomic DNA Sequences244
The Number of Protein-Coding Genes in an Organism’s Genome Is Not Directly Related to Its Biological Complexity245
Single Nucleotide Polymorphisms and Gene Copy-Number Variation Are Important Determinants of Differences Between Individuals of a Species246
6.6 Structural Organization of Eukaryotic Chromosomes247
Chromatin Exists in Extended and Condensed Forms248
Modifications of Histone Tails Control Chromatin Condensation and Function250
Nonhistone Proteins Provide a Structural Scaffold for Long Chromatin Loops254
Additional Nonhistone Proteins Regulate Transcription and Replication256
6.7 Morphology and Functional Elements of Eukaryotic Chromosomes257
Chromosome Number,Size,and Shape at Metaphase Are Species-Specific257
During Metaphase,Chromosomes Can Be Distinguished by Banding Patterns and Chromosome Painting258
Chromosome Painting and DNA Sequencing Reveal the Evolution of Chromosomes259
Interphase Polytene Chromosomes Arise by DNA Amplification260
Three Functional Elements Are Required for Replication and Stable Inheritance of Chromosomes261
Centromere Sequences Vary Greatly in Length263
Addition of Telomeric Sequences by Telomerase Prevents Shortening of Chromosomes263
7 TRANSCRIPTIONAL CONTROL OF GENE EXPRESSION269
7.1 Control of Gene Expression in Bacteria271
Transcription Initiation by Bacterial RNA Polymerase Requires Association with a Sigma Factor271
Initiation of Iac Operon Transcription Can Be Repressed and Activated271
Small Molecules Regulate Expression of Many Bacterial Genes via DNA-Binding Repressors and Activators273
Transcription Initiation from Some Promoters Requires Alternative Sigma Factors273
Transcription by σ54-RNA Polymerase Is Controlled by Activators That Bind Far from the Promoter274
Many Bacterial Responses Are Controlled by Two-Component Regulatory Systems275
7.2 Overview of Eukaryotic Gene Control and RNA Polymerases276
Regulatory Elements in Eukaryotic DNA Are Found Both Close to and Many Kilobases Away from Transcription Start Sites276
Three Eukaryotic Polymerases Catalyze Formation of Different RNAs278
The Largest Subunit in RNA Polymerase Ⅱ Has an Essential Carboxyl-Terminal Repeat279
RNA Polymerase Ⅱ Initiates Transcription at DNA Sequences Corresponding to the 5’ Cap of mRNAs280
7.3 Regulatory Sequences in Protein-Coding Genes282
The TATA Box,Initiators,and CpG Islands Function as Promoters in Eukaryotic DNA282
Promoter-Proximal Elements Help Regulate Eukaryotic Genes282
Distant Enhancers Often Stimulate Transcription by RNA Polymerase Ⅱ284
Most Eukaryotic Genes Are Regulated by Multiple Transcription-Control Elements285
7.4 Activators and Repressors of Transcription286
Footprinting and Gel-Shift Assays Detect Protein-DNA Interactions286
Activators Are Modular Proteins Composed of Distinct Functional Domains and Promote Transcription288
Repressors Inhibit Transcription and Are the Functional Converse of Activators290
DNA-Binding Domains Can Be Classified into Numerous Structural Types290
Structurally Diverse Activation and Repression Domains Regulate Transcription293
Transcription Factor Interactions Increase Gene-Control Options294
Multiprotein Complexes Form on Enhancers295
7.5 Transcription Initiation by RNA Polymerase Ⅱ296
General Transcription Factors Position RNA Polymerase Ⅱ at Start Sites and Assist in Initiation296
Sequential Assembly of Proteins Forms the Pol Ⅱ Transcription Preinitiation Complex in Vitro297
In Vivo Transcription Initiation by Pol Ⅱ Requires Additional Proteins298
7.6 Molecular Mechanisms of Transcription Repression and Activation299
Formation of Heterochromatin Silences Gene Expression at Telomeres,Near Centromeres,and in Other Regions299
Repressors Can Direct Histone Deacetylation and Methylation at Specific Genes303
Activators Can Direct Histone Acetylation and Methylation at Specific Genes305
Chromatin-Remodeling Factors Help Activate or Repress Transcription306
Histone Modifications Vary Greatly in Their Stabilities307
The Mediator Complex Forms a Molecular Bridge Between Activation Domains and Pol Ⅱ307
Transcription of Many Genes Requires Ordered Binding and Function of Activators and Co-activators308
The Yeast Two-Hybrid System Exploits Activator Flexibility to Detect cDNAs That Encode Interacting Proteins310
7.7 Regulation of Transcription-Factor Activity311
All Nuclear Receptors Share a Common Domain Structure312
Nuclear-Receptor Response Elements Contain Inverted or Direct Repeats313
Hormone Binding to a Nuclear Receptor Regulates Its Activity as a Transcription Factor313
7.8 Regulated Elongation and Termination of Transcription314
Transcription of the HIV Genome Is Regulated by an Antitermination Mechanism315
Promoter-Proximal Pausing of RNA Polymerase Ⅱ Occurs in Some Rapidly Induced Genes316
7.9 Other Eukaryotic Transcription Systems316
Transcription Initiation by Pol Ⅰ and Pol Ⅲ Is Analogous to That by Pol Ⅱ316
Mitochondrial and Chloroplast DNAs Are Transcribed by Organelle-Specific RNA Polymerases317
8 POST-TRANSCRIPTIONAL GENE CONTROL323
8.1 Processing of Eukaryotic Pre-mRNA325
The 5’ Cap Is Added to Nascent RNAs Shortly After Transcription Initiation325
A Diverse Set of Proteins with Conserved RNA-Binding Domains Associate with Pre-mRNAs326
Splicing Occurs at Short,Conserved Sequences in Pre-mRNAs via Two Transesterification Reactions329
During Splicing,snRNAs Base-Pair with Pre-mRNA330
Spliceosomes,Assembled from snRNPs and a Pre-mRNA,Carry Out Splicing330
Chain Elongation by RNA Polymerase Ⅱ Is Coupled to the Presence of RNA-Processing Factors333
SR Proteins Contribute to Exon Definition in Long Pre-mRNAs333
Self-Splicing Group Ⅱ Introns Provide Clues to the Evolution of snRNAs334
3’ Cleavage and Polyadenylation of Pre-mRNAs Are Tightly Coupled335
Nuclear Exonucleases Degrade RNA That Is Processed Out of Pre-mRNAs336
8.2 Regulation of Pre-mRNA Processing337
Alternative Splicing Is the Primary Mechanism for Regulating mRNA Processing337
A Cascade of Regulated RNA Splicing Controls Drosophila Sexual Differentiation338
Splicing Repressors and Activators Control Splicing at Alternative Sites339
RNA Editing Alters the Sequences of Some Pre-mRNAs340
8.3 Transport of mRNA Across the Nuclear Envelope341
Nuclear Pore Complexes Control Import and Exportfrom the Nucleus342
Pre-mRNAs in Spliceosomes Are Not Exported from the Nucleus345
HIV Rev Protein Regulates the Transport of Unspliced Viral mRNAs346
8.4 Cytoplasmic Mechanisms of Post-transcriptional Control347
Micro RNAs Repress Translation of Specific mRNAs347
RNA Interference Induces Degradation of Precisely Complementary mRNAs349
Cytoplasmic Polyadenylation Promotes Translation of Some mRNAs351
Degradation of mRNAs in the Cytoplasm Occurs by Several Mechanisms352
Protein Synthesis Can Be Globally Regulated353
Sequence-Specific RNA-Binding Proteins Control Specific mRNA Translation356
Surveillance Mechanisms Prevent Translation of Improperly Processed mRNAs357
Localization of mRNAs Permits Production of Proteins at Specific Regions Within the Cytoplasm357
8.5 Processing of rRNA and tRNA358
Pre-rRNA Genes Function as Nucleolar Organizers and Are Similar in All Eukaryotes359
Small Nucleolar RNAs Assist in Processing Pre-rRNAs360
Self-Splicing Group I Introns Were the First Examples of Catalytic RNA363
Pre-tRNAs Undergo Extensive Modification in the Nucleus363
Nuclear Bodies Are Functionally Specialized Nuclear Domains364
Part Ⅲ Cell Structure and Function371
9 VISUALIZING,FRACTIONATING,AND CULTURING CELLS371
9.1 Organelles of the Eukaryotic Cell372
The Plasma Membrane Has Many Common Functions in All Cells372
Endosomes Take Up Soluble Macromolecules from the Cell Exterior372
Lysosomes Are Acidic Organelles That Contain a Battery of Degradative Enzymes373
Peroxisomes Degrade Fatty Acids and Toxic Compounds374
The Endoplasmic Reticulum Is a Network of Interconnected Internal Membranes375
The Golgi Complex Processes and Sorts Secreted and Membrane Proteins376
Plant Vacuoles Store Small Molecules and Enable a Cell to Elongate Rapidly377
The Nucleus Contains the DNA Genome,RNA Synthetic Apparatus,and a Fibrous Matrix378
Mitochondria Are the Principal Sites of ATP Production in Aerobic Non photosynthetic Cells378
Chloroplasts Contain Internal Compartments in Which Photosynthesis Takes Place379
9.2 Light Microscopy:Visualizing Cell Structure and Localizing Proteins Within Cells380
The Resolution of the Light Microscope Is About 0.2 μm381
Phase-Contrast and Differential Interference Contrast Microscopy Visualize Unstained Living Cells381
Fluorescence Microscopy Can Localize and Quantify Specific Molecules in Live Cells382
Imaging Subcellular Details Often Requires that the Samples Be Fixed,Sectioned,and Stained384
Immunofluorescence Microscopy Can Detect Specific Proteins in Fixed Cells385
Confocal and Deconvolution Microscopy Enable Visualization of Three-Dimensional Objects386
Graphics and Informatics Have Transformed Modern Microscopy387
9.3 Electron Microscopy:Methods and Applications388
Resolution of Transmission Electron Microscopy is Vastly Greater Than That of Light Microscopy388
Cryoelectron Microscopy Allows Visualization of Particles Without Fixation or Staining389
Electron Microscopy of Metal-Coated Specimens Can Reveal Surface Features of Cells and Their Components390
9.4 Purification of Cell Organelles391
Disruption of Cells Releases Their Organelles and Other Contents391
Centrifugation Can Separate Many Types of Organelles392
Organelle-Specific Antibodies Are Useful in Preparing Highly Purified Organelles393
9.5 Isolation,Culture,and Differentiation of Metazoan Cells394
Flow Cytometry Separates Different Cell Types394
Culture of Animal Cells Requires Nutrient-Rich Media and Special Solid Surfaces395
Primary Cell Cultures Can Be Used to Study Cell Differentiation396
Primary Cell Cultures and Cell Strains Have a Finite Life Span396
Transformed Cells Can Grow Indefinitely in Culture397
Some Cell Lines Undergo Differentiation in Culture398
Hybrid Cells Called Hybridomas Produce Abundant Monoclonal Antibodies400
HAT Medium Is Commonly Used to Isolate Hybrid Cells402
CLASSIC EXPERIMENT 9.1 Separating Organelles407
10 BIOMEMBRANE STRUCTURE409
10.1 Biomembranes:Lipid Composition and Structural Organization411
Phospholipids Spontaneously Form Bilayers411
Phospholipid Bilayers Form a Sealed Compartment Surrounding an Internal Aqueous Space411
Biomembranes Contain Three Principal Classes of Lipids415
Most Lipids and Many Proteins Are Laterally Mobile in Biomembranes416
Lipid Composition Influences the Physical Properties of Membranes418
Lipid Composition Is Different in the Exoplasmic and Cytosolic Leaflets419
Cholesterol and Sphingolipids Cluster with Specific Proteins in Membrane Microdomains420
10.2 Biomembranes:Protein Components and Basic Functions421
Proteins Interact with Membranes in Three Different Ways421
Most Transmembrane Proteins Have Membrane-Spanning α Helices422
Multiple β Strands in Porins Form Membrane-Spanning “Barrels”424
Covalently Attached Hydrocarbon Chains Anchor Some Proteins to Membranes424
All Transmembrane Proteins and Glycolipids Are Asymmetrically Oriented in the Bilayer426
Lipid-Binding Motifs Help Target Peripheral Proteins to the Membrane427
Proteins Can Be Removed from Membranes by Detergents or High-Salt Solutions427
10.3 Phospholipids,Sphingolipids,and Cholesterol:Synthesis and Intracellular Movement429
Fatty Acids Synthesis Is Mediated by Several Important Enzymes430
Small Cytosolic Proteins Facilitate Movement of Fatty Acids430
Incorporation of Fatty Acids into Membrane Lipids Takes Place on Organelle Membranes431
Flippases Move Phospholipids from One Membrane Leaflet to the Opposite Leaflet431
Cholesterol Is Synthesized by Enzymes in the Cytosol and ER Membrane432
Cholesterol and Phospholipids Are Transported Between Organelles by Several Mechanisms433
11 TRANSMEMBRANE TRANSPORT OF IONS AND SMALL MOLECULES437
11.1 Overview of Membrane Transport438
Only Small Hydrophobic Molecules Cross Membranes by Simple Diffusion438
Membrane Proteins Mediate Transport of Most Molecules and All Ions Across Biomembranes439
11.2 Uniport Transport of Glucose and Water441
Several Features Distinguish Uniport Transport from Simple Diffusion441
GLUT1 Uniporter Transports Glucose into Most Mammalian Cells442
The Human Genome Encodes a Family of Sugar-Transporting GLUT Proteins443
Transport Proteins Can Be Enriched Within Artificial Membranes and Cells443
Osmotic Pressure Causes Water to Move Across Membranes444
Aquaporins Increase the Water Permeability of Cell Membranes444
11.3 ATP-Powered Pumps and the Intracellular Ionic Environment447
Different Classes of Pumps Exhibit Characteristic Structural and Functional Properties447
ATP-Powered Ion Pumps Generate and Maintain Ionic Gradients Across Cellular Membranes448
Muscle Relaxation Depends on Ca 2+ ATPases That Pump Ca 2+ from the Cytosol into the Sarcoplasmic Reticulum449
Calmodulin Regulates the Plasma Membrane Ca 2+ Pumps That Control Cytosolic Ca 2+Concentrations451
Na+/K+ ATPase Maintains the Intracellular Na+and K+ Concentrations in Animal Cells452
V-Class H+ ATPases Maintain the Acidity of Lysosomes and Vacuoles453
Bacterial Permeases Are ABC Proteins That Import a Variety of Nutrients from the Environment454
The Approximately 50 Mammalian ABC Transporters Play Diverse and Important Roles in Cell and Organ Physiology455
Certain ABC Proteins “Flip” Phospholipids and Other Lipid-Soluble Substrates from One Membrane Leaflet to the Opposite Leaflet456
11.4 Nongated Ion Channels and the Resting Membrane Potential458
Selective Movement of Ions Creates a Transmembrane Electric Potential Difference458
The Membrane Potential in Animal Cells Depends Largely on Potassium Ion Movements Through Open Resting K+ Channels460
Ion Channels Contain a Selectivity Filter Formed from Conserved Transmembrane Segments461
Patch Clamps Permit Measurement of Ion Movements Through Single Channels463
Novel Ion Channels Can Be Characterized by a Combination of Oocyte Expression and Patch Clamping464
Na+ Entry into Mammalian Cells Has a Negative Change in Free Energy(△G)464
11.5 Cotransport by Symporters and Antiporters465
Na+-Linked Symporters Import Amino Acids and Glucose into Animal Cells Against High Concentration Gradients466
Bacterial Symporter Structure Reveals the Mechanism of Substrate Binding467
Na+-Linked Ca 2+ Antiporter Exports Ca 2+ from Cardiac Muscle Cells468
Several Cotransporters Regulate Cytosolic pH468
A Putative Cation Exchange Protein Plays a Key Role in Evolution of Human Skin Pigmentation469
Numerous Transport Proteins Enable Plant Vacuoles to Accumulate Metabolites and Ions469
11.6 Transepithelial Transport470
Multiple Transport Proteins Are Needed to Move Glucose and Amino Acids Across Epithelia471
Simple Rehydration Therapy Depends on the Osmotic Gradient Created by Absorption of Glucose and Na+471
Parietal Cells Acidify the Stomach Contents While Maintaining a Neutral Cytosolic pH472
CLASSIC EXPERIMENT 11.1 Stumbling Upon Active Transport477
12 CELLULAR ENERGETICS479
12.1 First Steps of Glucose and Fatty Acid Catabolism:Glycolysis and the Citric Acid Cycle480
During Glycolysis(Stage Ⅰ),Cytosolic Enzymes Convert Glucose to Pyruvate481
The Rate of Glycolysis Is Adjusted to Meet the Cell’s Need for ATP483
Glucose Is Fermented Under Anaerobic Conditions485
Under Aerobic Conditions,Mitochondria Efficiently Oxidize Pyruvate and Generate ATP(Stages Ⅱ-Ⅳ)485
Mitochondria Are Dynamic Organelles with Two Structurally and Functionally Distinct Membranes485
In Stage Ⅱ,Pyruvate Is Oxidized to CO2 and High-Energy Electrons Stored in Reduced Coenzymes487
Transporters in the Inner Mitochondrial Membrane Help Maintain Appropriate Cytosolic and Matrix Concentrations of NAD+ and NADH489
Mitochondrial Oxidation of Fatty Acids Generates ATP491
Peroxisomal Oxidation of Fatty Acids Generates No ATP491
12.2 The Electron Transport Chain and Generation of the Proton-Motive Force493
Stepwise Electron Transport Efficiently Releases the Energy Stored in NADH and FADH2493
Electron Transport in Mitochondria Is Coupled to Proton Pumping493
Electrons Flow from FADH2 and NADH to O2 Through Four Multiprotein Complexes494
Reduction Potentials of Electron Carriers Favor Electron Flow from NADH to O2499
Experiments Using Purified Complexes Established the Stoichiometry of Proton Pumping499
The Q Cycle Increases the Number of Protons Translocated as Electrons Flow Through Complex Ⅲ500
The Proton-Motive Force in Mitochondria Is Due Largely to a Voltage Gradient Across the Inner Membrane502
Toxic By-products of Electron Transport Can Damage Cells502
12.3 Harnessing the Proton-Motive Force for Energy-Requiring Processes503
The Mechanism of ATP Synthesis Is Shared Among Bacteria,Mitochondria,and Chloroplasts505
ATP Synthase Comprises Two Multiprotein Complexes Termed F0 and F1505
Rotation of the F1 γ Subunit,Driven by Proton Movement Through F0,Powers ATP Synthesis506
ATP-ADP Exchange Across the Inner Mitochondrial Membrane Is Powered by the Proton-Motive Force509
Rate of Mitochondrial Oxidation Normally Depends on ADP Levels510
Brown-Fat Mitochondria Use the Proton-Motive Force to Generate Heat510
12.4 Photosynthesis and Light-Absorbing Pigments511
Thylakoid Membranes in Chloroplasts Are the Sites of Photosynthesis in Plants511
Three of the Four Stages in Photosynthesis Occur Only During Illumination511
Each Photon of Light Has a Defined Amount of Energy513
Photosystems Comprise a Reaction Center and Associated Light-Harvesting Complexes514
Photoelectron Transport from Energized Reaction-Center Chlorophyll a Produces a Charge Separation514
Internal Antenna and Light-Harvesting Complexes Increase the Efficiency of Photosynthesis515
12.5 Molecular Analysis of Photosystems517
The Single Photosystem of Purple Bacteria Generates a Proton-Motive Force but No O2517
Linear Electron Flow Through Both Plant Photosystems,PSII and PSI,Generates a Proton-Motive Force,O2,and NADPH519
An Oxygen-Evolving Complex Is Located on the Luminal Surface of the PSII Reaction Center520
Cells Use Multiple Mechanisms to Protect Against Damage from Reactive Oxygen Species During Photoelectron Transport521
Cyclic Electron Flow Through PSI Generates a Proton-Motive Force but No NADPH or O2522
Relative Activities of Photosystems Ⅰ and Ⅱ Are Regulated523
12.6 CO2 Metabolism During Photosynthesis524
Rubisco Fixes CO2 in the Chloroplast Stroma525
Synthesis of Sucrose Using Fixed CO2 Is Completed in the Cytosol525
Light and Rubisco Activase Stimulate CO2 Fixation525
Photorespiration,Which Competes with Photosynthesis,Is Reduced in Plants That Fix CO2 by the C4 Pathway527
13 MOVING PROTEINS INTO MEMBRANES AND ORGANELLES533
13.1 Translocation of Secretory Proteins Across the ER Membrane535
A Hydrophobic N-Terminal Signal Sequence Targets Nascent Secretory Proteins to the ER536
Cotranslational Translocation Is Initiated by Two GTP-Hydrolyzing Proteins537
Passage of Growing Polypeptides Through the Translocon Is Driven by Energy Released During Translation539
ATP Hydrolysis Powers Post-translational Translocation of Some Secretory Proteins in Yeast540
13.2 Insertion of Proteins into the ER Membrane542
Several Topological Classes of Integral Membrane Proteins Are Synthesized on the ER543
Internal Stop-Transfer and Signal-Anchor Sequences Determine Topology of Single-Pass Proteins544
Multipass Proteins Have Multiple Internal Topogenic Sequences546
A Phospholipid Anchor Tethers Some Cell-Surface Proteins to the Membrane547
The Topology of a Membrane Protein Often Can Be Deduced from Its Sequence547
13.3 Protein Modifications,Folding,and Quality Control in the ER549
A Preformed N-Linked Oligosaccharide Is Added to Many Proteins in the Rough ER550
Oligosaccharide Side Chains May Promote Folding and Stability of Glycoproteins552
Disulfide Bonds Are Formed and Rearranged by Proteins in the ER Lumen552
Chaperones and Other ER Proteins Facilitate Folding and Assembly of Proteins552
Improperly Folded Proteins in the ER Induce Expression of Protein-Folding Catalysts555
Unassembled or Misfolded Proteins in the ER Are Often Transported to the Cytosol for Degradation556
13.4 Sorting of Proteins to Mitochondria and Chloroplasts557
Amphipathic N-Terminal Signal Sequences Direct Proteins to the Mitochondrial Matrix558
Mitochondrial Protein Import Requires Outer-Membrane Receptors and Translocons in Both Membranes558
Studies with Chimeric Proteins Demonstrate Important Features of Mitochondrial Import560
Three Energy Inputs Are Needed to Import Proteins into Mitochondria561
Multiple Signals and Pathways Target Proteins to Submitochondrial Compartments561
Targeting of Chloroplast Stromal Proteins Is Similar to Import of Mitochondrial Matrix Proteins565
Proteins Are Targeted to Thylakoids by Mechanisms Related to Translocation Across the Bacterial Inner Membrane565
13.5 Sorting of Peroxisomal Proteins567
Cytosolic Receptor Targets Proteins with an SKL Sequence at the C-Terminus into the Peroxisomal Matrix567
Peroxisomal Membrane and Matrix Proteins Are Incorporated by Different Pathways568
13.6 Transport into and out of the Nucleus569
Large and Small Molecules Enter and Leave the Nucleus via Nuclear Pore Complexes570
Importins Transport Proteins Containing Nuclear- Localization Signals into the Nucleus571
Exportins Transport Proteins Containing Nuclear-ExportSignals out of the Nucleus573
Most mRNAs Are Exported from the Nucleus by a Ran-Independent Mechanism573
14 VESICULAR TRAFFIC,SECRETION,AND ENDOCYTOSIS579
14.1 Techniques for Studying the Secretory Pathway580
Transport of a Protein Through the Secretory Pathway Can Be Assayed in Living Cells582
Yeast Mutants Define Major Stages and Many Components in Vesicular Transport584
Cell-Free Transport Assays Allow Dissection of Individual Steps in Vesicular Transport585
14.2 Molecular Mechanisms of Vesicular Traffic586
Assembly of a Protein Coat Drives Vesicle Formation and Selection of Cargo Molecules586
A Conserved Set of GTPase Switch Proteins Controls Assembly of Different Vesicle Coats587
Targeting Sequences on Cargo Proteins Make Specific Molecular Contacts with Coat Proteins588
Rab GTPases Control Docking of Vesicles on Target Membranes589
Paired Sets of SNARE Proteins Mediate Fusion of Vesicles with Target Membranes591
Dissociation of SNARE Complexes After Membrane Fusion Is Driven by ATP Hydrolysis591
14.3 Early Stages of the Secretory Pathway592
COPII Vesicles Mediate Transport from the ER to the Golgi592
COPI Vesicles Mediate Retrograde Transport within the Golgi and from the Golgi to the ER594
Anterograde Transport Through the Golgi Occurs by Cisternal Maturation595
14.4 Later Stages of the Secretory Pathway597
Vesicles Coated with Clathrin and/or Adapter Proteins Mediate Several Transport Steps598
Dynamin Is Required for Pinching Off of Clathrin Vesicles599
Mannose 6-Phosphate Residues Target Soluble Proteins to Lysosomes600
Study of Lysosomal Storage Diseases Revealed Key Components of the Lysosomal Sorting Pathway602
Protein Aggregation in the trans-Golgi May Function in Sorting Proteins to Regulated Secretory Vesicles602
Some Proteins Undergo Proteolytic Processing After Leaving the trans-Golgi603
Several Pathways Sort Membrane Proteins to the Apical or Basolateral Region of Polarized Cells604
14.5 Receptor-Mediated Endocytosis606
Cells Take Up Lipids from the Blood in the Form of Large,Well-Defined Lipoprotein Complexes606
Receptors for Low-Density Lipoprotein and Other Ligands Contain Sorting Signals That Target Them for Endocytosis608
The Acidic pH of Late Endosomes Causes Most Receptor-Ligand Complexes to Dissociate610
The Endocytic Pathway Delivers Iron to Cells without Dissociation of the Receptor-Transferrin Complex in Endosomes611
14.6 Directing Membrane Proteins and Cytosolic Materials to the Lysosome612
Multivesicular Endosomes Segregate Membrane Proteins Destined for the Lysosomal Membrane from Proteins Destined for Lysosomal Degradation612
Retroviruses Bud from the Plasma Membrane by a Process Similar to Formation of Multivesicular Endosomes614
CLASSIC EXPERIMENT 14.1 Following a Protein Out of the Cell621
15 CELL SIGNALING Ⅰ:SIGNAL TRANSDUCTION AND SHORT-TERM CELLULAR RESPONSES623
15.1 From Extracellular Signal to Cellular Response625
Signaling Cells Produce and Release Signaling Molecules625
Signaling Molecules Can Act Locally or at a Distance625
Binding of Signaling Molecules Activates Receptors on Target Cells626
15.2 Studying Cell-Surface Receptors627
Receptor Proteins Bind Ligands Specifically627
The Dissociation Constant Is a Measure of the Affinity of a Receptor for Its Ligand628
Binding Assays Are Used to Detect Receptors and Determine Their Affinities for Ligands628
Maximal Cellular Response to a Signaling Molecule Usually Does Not Require Activation of All Receptors629
Sensitivity of a Cell to External Signals Is Determined by the Number of Surface Receptors and Their Affinity for Ligand631
Receptors Can Be Purified by Affinity Techniques631
Receptors Are Frequently Expressed from Cloned Genes631
15.3 Highly Conserved Components of Intracellular Signal-Transduction Pathways632
GTP-Binding Proteins Are Frequently Used As On/Off Switches633
Protein Kinases and Phosphatases are Employed in Virtually All Signaling Pathways634
Second Messengers Carry and Amplify Signals from Many Receptors634
15.4 General Elements of G Protein-Coupled Receptor Systems635
G Protein-Coupled Receptors Are a Large and Diverse Family with a Common Structure and Function635
G Protein-Coupled Receptors Activate Exchange of GTP for GDP on the α Subunit of a Trimeric G Protein637
Different G Proteins Are Activated by Different GPCRs and In Turn Regulate Different Effector Proteins639
15.5 G Protein-Coupled Receptors That Regulate Ion Channels640
Acetylcholine Receptors in the Heart Muscle Activate a G Protein That Opens K+ Channels641
Light Activates Gαt-Coupled Rhodopsins641
Activation of Rhodopsin Induces Closing of cGMP-Gated Cation Channels642
Rod Cells Adapt to Varying Levels of Ambient Light Because of Opsin Phosphorylation and Binding of Arrestin644
15.6 G Protein-Coupled Receptors That Activate or Inhibit Adenylyl Cyclase646
Adenylyl Cyclase Is Stimulated and Inhibited by Different Receptor-Ligand Complexes646
Structural Studies Established How Gαs·GTP Binds to and Activates Adenylyl Cyclase646
cAMP Activates Protein Kinase A by Releasing Catalytic Subunits647
Glycogen Metabolism Is Regulated by Hormone-Induced Activation of Protein Kinase A648
cAMP-Mediated Activation of Protein Kinase A Produces Diverse Responses in Different Cell Types649
Signal Amplification Commonly Occurs in Many Signaling Pathways650
Several Mechanisms Down-Regulate Signaling from G Protein-Coupled Receptors651
Anchoring Proteins Localize Effects of cAMP to Specific Regions of the Cell652
15.7 G Protein-Coupled Receptors That Activate Phospholipase C653
Phosphorylated Derivatives of Inositol Are Important Second Messengers654
Calcium Ion Release from the Endoplasmic Reticulum is Triggered by IP3654
The Ca 2+/Calmodulin Complex Mediates Many Cellular Responses to External Signals655
Diacylglycerol(DAG) Activates Protein Kinase C,Which Regulates Many Other Proteins656
Signal-Induced Relaxation of Vascular Smooth Muscle Is Mediated by cGMP-Activated Protein Kinase G656
15.8 Integrating Responses of Cells to Environmental Influences657
Integration of Multiple Second Messengers Regulates Glycogenolysis657
Insulin and Glucagon Work Together to Maintain a Stable Blood Glucose Level658
CLASSIC EXPERIMENT 15.1 The Infancy of Signal Transduction—GTP Stimulation of cAMP Synthesis663
16 CELL-SIGNALING Ⅱ:SIGNALING PATHWAYS THAT CONTROL GENE ACTIVITY665
16.1 TGFβ Receptors and the Direct Activation of Smads668
A TGFβ Signaling Molecule Is Formed by Cleavage of an Inactive Precursor668
Radioactive Tagging Was Used to Identify TGFβ Receptors669
Activated TGFβ Receptors Phosphorylate Smad Transcription Factors670
Negative Feedback Loops Regulate TGFβ/Smad Signaling671
Loss of TGFβ Signaling Plays a Key Role in Cancer671
16.2 Cytokine Receptors and the JAK/STAT Pathway672
Cytokines Influence Development of Many Cell Types672
Cytokine Receptors Have Similar Structures and Activate Similar Signaling Pathways673
JAK Kinases Activate STAT Transcription Factors674
Complementation Genetics Revealed That JAK and STAT Proteins Transduce Cytokine Signals677
Signaling from Cytokine Receptors Is Regulated by Negative Signals678
Mutant Erythropoietin Receptor That Cannot Be Turned Off Leads to Increased Numbers of Erythrocytes679
16.3 Receptor Tyrosine Kinases679
Ligand Binding Leads to Phosphorylation and Activation of Intrinsic Kinase in RTKs680
Overexpression of HER2,a Receptor Tyrosine Kinase,Occurs in Some Breast Cancers680
Conserved Domains Are Important for Binding Signal-Transduction Proteins to Activated Receptors682
Down-regulation of RTK Signaling Occurs by Endocytosis and Lysosomal Degradation683
16.4 Activation of Ras and MAP Kinase Pathways684
Ras,a GTPase Switch Protein,Cycles Between Active and Inactive States685
Receptor Tyrosine Kinases Are Linked to Ras by Adapter Proteins685
Genetic Studies in Drosophila Identified Key Signal-Transducing Proteins in the Ras/MAP Kinase Pathway685
Binding of Sos Protein to Inactive Ras Causes a Conformational Change That Activates Ras687
Signals Pass from Activated Ras to a Cascade of Protein Kinases688
MAP Kinase Regulates the Activity of Many Transcription Factors Controlling Early-Response Genes690
G Protein-Coupled Receptors Transmit Signals to MAP Kinase in Yeast Mating Pathways691
Scaffold Proteins Separate Multiple MAP Kinase Pathways in Eukaryotic Cells692
The Ras/MAP Kinase Pathway Can Induce Diverse Cellular Responses693
16.5 Phosphoinositides as Signal Transducers694
Phospholipase Cγ Is Activated by Some RTKs and Cytokine Receptors694
Recruitment of PI-3 Kinase to Hormone-Stimulated Receptors Leads to Synthesis of Phosphorylated Phosphatidylinositols694
Accumulation of PI 3-Phosphates in the Plasma Membrane Leads to Activation of Several Kinases695
Activated Protein Kinase B Induces Many Cellular Responses696
The PI-3 Kinase Pathway Is Negatively Regulated by PTEN Phosphatase697
16.6 Activation of Gene Transcription by Seven-Spanning Cell-Surface Receptors697
CREB Links cAMP and Protein Kinase A to Activation of Gene Transcription698
GPCR-Bound Arrestin Activates Several Kinase Cascades698
Wnt Signals Trigger Release of a Transcription Factor from Cytosolic Protein Complex699
Hedgehog Signaling Relieves Repression of Target Genes700
16.7 Pathways That Involve Signal-Induced Protein Cleavage703
Degradation of an Inhibitor Protein Activates the NF-κB Transcription Factors703
Ligand-Activated Notch Is Cleaved Twice,Releasing a Transcription Factor705
Matrix Metal loproteases Catalyze Cleavage of Many Signaling Proteins from the Cell Surface706
Inappropriate Cleavage of Amyloid Precursor Protein Can Lead to Alzheimer’s Disease706
Regulated Intramembrane Proteolysis of SREBP Releases a Transcription Factor That Acts to Maintain Phospholipid and Cholesterol Levels707
17 CELL ORGANIZATION AND MOVEMENT Ⅰ:MICROFILAMENTS713
17.1 Microfilaments and Actin Structures716
Actin Is Ancient,Abundant,and Highly Conserved717
G-Actin Monomers Assemble into Long,Helical F-Actin Polymers717
F-Actin Has Structural and Functional Polarity718
17.2 Dynamics of Actin Filaments718
Actin Polymerization in Vitro Proceeds in Three Steps719
Actin Filaments Grow Faster at(+) Ends Than at(-) Ends720
Actin Filament Treadmilling Is Accelerated by Profilin and Cofilin721
Thymosin-β4 Provides a Reservoir of Actin for Polymerization722
Capping Proteins Block Assembly and Disassembly at Actin Filament Ends722
17.3 Mechanisms of Actin Filament Assembly723
Formins Assemble Unbranched Filaments723
The Arp2/3 Complex Nucleates Branched Filament Assembly724
Intracellular Movements Can Be Powered by Actin Polymerization726
Toxins That Perturb the Pool of Actin Monomers Are Useful for Studying Actin Dynamics726
17.4 Organization of Actin-Based Cellular Structures728
Cross-Linking Proteins Organize Actin Filaments into Bundles or Networks728
Adaptor Proteins Link Actin Filaments to Membranes728
17.5 Myosins:Actin-Based Motor Proteins731
Myosins Have Head,Neck,and Tail Domains with Distinct Functions732
Myosins Make Up a Large Family of Mechanochemical Motor Proteins733
Conformational Changes in the Myosin Head Couple ATP Hydrolysis to Movement736
Myosin Heads Take Discrete Steps Along Actin Filaments736
Myosin V Walks Hand Over Hand Down an Actin Filament737
17.6 Myosin-Powered Movements738
Myosin Thick Filaments and Actin Thin Filaments in Skeletal Muscle Slide Past One Another During Contraction738
Skeletal Muscle Is Structured by Stabilizing and Scaffolding Proteins740
Contraction of Skeletal Muscle Is Regulated by Ca 2+ and Actin-Binding Proteins740
Actin and Myosin Ⅱ Form Contractile Bundles in Nonmuscle Cells741
Myosin-Dependent Mechanisms Regulate Contraction in Smooth Muscle and Nonmuscle Cells742
Myosin-V-Bound Vesicles Are Carried Along Actin Filaments743
17.7 Cell Migration:Signaling and Chemotaxis745
Cell Migration Coordinates Force Generation with Cell Adhesion and Membrane Recycling745
The Small GTP-Binding Proteins Cdc42,Rac,and Rho Control Actin Organization747
Cell Migration Involves the Coordinate Regulation of Cdc42,Rac,and Rho748
Migrating Cells Are Steered by Chemotactic Molecules750
Chemotactic Gradients Induce Altered Phosphoinositide Levels Between the Front and Back of a Cell750
CLASSIC EXPERIMENT 17.1 Looking at Muscle Contraction755
18 CELL ORGANIZATION AND MOVEMENT Ⅱ:MICROTUBULES AND INTERMEDIATE FILAMENTS757
18.1 Microtubule Structure and Organization758
Microtubule Walls Are Polarized Structures Built from αβ-Tubulin Dimers758
Microtubules Are Assembled from MTOCs to Generate Diverse Organizations760
18.2 Microtubule Dynamics762
Microtubules Are Dynamic Structures Due to Kinetic Differences at Their Ends763
Individual Microtubules Exhibit Dynamic Instability763
Localized Assembly and “Search-and-Capture” Help Organize Microtubules766
Drugs Affecting Tubulin Polymerization Are Useful Experimentally and to Treat Diseases766
18.3 Regulation of Microtubule Structure and Dynamics767
Microtubules Are Stabilized by Side- and End-Binding Proteins767
Microtubules Are Disassembled by End Binding and Severing Proteins768
18.4 Kinesins and Dyneins:Microtubule- Based Motor Proteins769
Organelles in Axons Are Transported Along Microtubules in Both Directions769
Kinesin-1 Powers Anterograde Transport of Vesicles Down Axons Toward the(+) End of Microtubules770
Kinesins Form a Large Protein Family with Diverse Functions771
Kinesin-1 Is a Highly Processive Motor772
Dynein Motors Transport Organelles Toward the(-)End of Microtubules774
Kinesins and Dyneins Cooperate in the Transport of Organelles Throughout the Cell775
18.5 Cilia and Flagella:Microtubule-Based Surface Structures777
Eukaryotic Cilia and Flagella Contain Long Doublet Microtubules Bridged by Dynein Motors777
Ciliary and Flagellar Beating Are Produced by Controlled Sliding of Outer Doublet Microtubules778
Intraflagellar Transport Moves Material Up and Down Cilia and Flagella779
Defects in Intraflagellar Transport Cause Disease by Affecting Sensory Primary Cilia780
18.6 Mitosis781
Mitosis Can Be Divided into Six Phases782
Centrosomes Duplicate Early in the Cell Cycle in Preparation for Mitosis783
The Mitotic Spindle Contains Three Classes of Microtubules784
Microtubule Dynamics Increases Dramatically in Mitosis784
Microtubules Treadmill During Mitosis785
The Kinetochore Captures and Helps Transport Chromosomes786
Duplicated Chromosomes Are Aligned by Motors and Treadmilling Microtubules788
Anaphase A Moves Chromosomes to Poles by Microtubule Shortening789
Anaphase B Separates Poles by the Combined Action of Kinesins and Dynein789
Additional Mechanisms Contribute to Spindle Formation789
Cytokinesis Splits the Duplicated Cell in Two789
Plant Cells Reorganize Their Microtubules and Build a New Cell Wall in Mitosis790
18.7 Intermediate Filaments791
Intermediate Filaments Are Assembled from Subunit Dimers792
Intermediate Filaments Proteins Are Expressed in a Tissue-Specific Manner792
Intermediate Filaments Are Dynamic795
Defects in Lamins and Keratins Cause Many Diseases795
18.8 Coordination and Cooperation between Cytoskeletal Elements796
Intermediate Filament-Associated Proteins Contribute to Cellular Organization796
Microfilaments and Microtubules Cooperate to Transport Melanosomes796
Cdc42 Coordinates Microtubules and Microfilaments During Cell Migration797
19 INTEGRATING CELLS INTO TISSUES801
19.1 Cell-Cell and Cell-Matrix Adhesion:An Overview803
Cell-Adhesion Molecules Bind to One Another and to Intracellular Proteins803
The Extracellular Matrix Participates in Adhesion,Signaling,and Other Functions805
The Evolution of Multifaceted Adhesion Molecules Enabled the Evolution of Diverse Animal Tissues807
19.2 Cell-Cell and Cell-ECM Junctions and Their Adhesion Molecules808
Epithelial Cells Have Distinct Apical,Lateral,and Basal Surfaces808
Three Types of Junctions Mediate Many Cell-Cell and Cell-ECM Interactions809
Cadherins Mediate Cell-Cell Adhesions in Adherens Junctions and Desmosomes810
Tight Junctions Seal Off Body Cavities and Restrict Diffusion of Membrane Components814
Integrins Mediate Cell-ECM Adhesions in Epithelial Cells816
Gap Junctions Composed of Connexins Allow Small Molecules to Pass Directly Between Adjacent Cells817
19.3 The Extracellular Matrix Ⅰ:The Basal Lamina820
The Basal Lamina Provides a Foundation for Assembly of Cells into Tissues820
Laminin,a Multiadhesive Matrix Protein,Helps Cross-link Components of the Basal Lamina821
Sheet-Forming Type Ⅳ Collagen Is a Major Structural Component of the Basal Lamina821
Perlecan,a Proteoglycan,Cross-links Components of the Basal Lamina and Cell-Surface Receptors824
19.4 The Extracellular Matrix Ⅱ:Connective and Other Tissues825
Fibrillar Collagens Are the Major Fibrous Proteins in the ECM of Connective Tissues825
Fibrillar Collagen Is Secreted and Assembled into Fibrils Outside of the Cell826
Type Ⅰ and Ⅱ Collagens Associate with Nonfibrillar Collagens to Form Diverse Structures826
Proteoglycans and Their Constituent GAGs Play Diverse Roles in the ECM827
Hyaluronan Resists Compression,Facilitates Cell Migration,and Gives Cartilage Its Gel-like Properties829
Fibronectins Interconnect Cells and Matrix,Influencing Cell Shape,Differentiation,and Movement830
19.5 Adhesive Interactions in Motile and Nonmotile Cells833
Integrins Relay Signals Between Cells and Their Three-Dimensional Environment833
Regulation of Integrin-Mediated Adhesion and Signaling Controls Cell Movement834
Connections Between the ECM and Cytoskeleton Are Defective in Muscular Dystrophy835
IgCAMs Mediate Cell-Cell Adhesion in Neuronal and Other Tissues836
Leukocyte Movement into Tissues Is Orchestrated by a Precisely Timed Sequence of Adhesive Interactions837
19.6 Plant Tissues839
The Plant Cell Wall Is a Laminate of Cellulose Fibrils in a Matrix of Glycoproteins840
Loosening of the Cell Wall Permits Plant Cell Growth840
Plasmodesmata Directly Connect the Cytosols of Adjacent Cells in Higher Plants840
Only a Few Adhesive Molecules Have Been Identified in Plants841
Part Ⅳ Cell Growth and Development847
20 REGULATING THE EUKARYOTIC CELL CYCLE847
20.1 Overview of the Cell Cycle and Its Control849
The Cell Cycle Is an Ordered Series of Events Leading to Cell Replication849
Regulated Protein Phosphorylation and Degradation Control Passage Through the Cell Cycle849
Diverse Experimental Systems Have Been Used to Identify and Isolate Cell-Cycle Control Proteins851
20.2 Control of Mitosis by Cyclins and MPF Activity853
Maturation-Promoting Factor(MPF) Stimulates Meiotic Maturation in Oocytes and Mitosis in Somatic Cells854
Mitotic Cyclin Was First Identified in Early Sea Urchin Embryos856
Cyclin B Levels and Kinase Activity of Mitosis-Promoting Factor(MPF) Change Together in Cycling Xenopus Egg Extracts856
Anaphase-Promoting Complex(APC/C) Controls Degradation of Mitotic Cyclins and Exit from Mitosis858
20.3 Cyclin-Dependent Kinase Regulation During Mitosis859
MPF Components Are Conserved Between Lower and Higher Eukaryotes860
Phosphorylation of the CDK Subunit Regulates the Kinase Activity of MPF861
Conformational Changes Induced by Cyclin Binding and Phosphorylation Increase MPF Activity862
20.4 Molecular Mechanisms for Regulating Mitotic Events864
Phosphorylation of Nuclear Lamins and Other Proteins Promotes Early Mitotic Events864
Unlinking of Sister Chromatids Initiates Anaphase867
Chromosome Decondensation and Reassembly of the Nuclear Envelope Depend on Dephosphorylation of MPF Substrates870
20.5 Cyclin-CDK and Ubiquitin-Protein Ligase Control of S phase872
A Cyclin-Dependent Kinase(CDK) Is Critical for S-Phase Entry in S.cerevisiae872
Three G1 Cyclins Associate with S.cerevisiae CDK to Form S-Phase-Promoting Factors874
Degradation of the S-Phase Inhibitor Triggers DNA Replication876
Multiple Cyclins Regulate the Kinase Activity of S.cerevisiae CDK During Different Cell-Cycle Phases877
Replication at Each Origin Is Initiated Only Once During the Cell Cycle877
20.6 Cell-Cycle Control in Mammalian Cells879
Mammalian Restriction Point Is Analogous to START in Yeast Cells880
Multiple CDKs and Cyclins Regulate Passage of Mammalian Cells Through the Cell Cycle881
Regulated Expression of Two Classes of Genes Returns G0 Mammalian Cells to the Cell Cycle881
Passage Through the Restriction Point Depends on Phosphorylation of the Tumor-Suppressor Rb Protein882
Cyclin A Is Required for DNA Synthesis and CDK1 for Entry into Mitosis883
Two Types of Cyclin-CDK Inhibitors Contribute to Cell-Cycle Control in Mammals883
20.7 Checkpoints in Cell-Cycle Regulation884
The Presence of Unreplicated DNA Prevents Entry into Mitosis888
Improper Assembly of the Mitotic Spindle Prevents the Initiation of Anaphase888
Proper Segregation of Daughter Chromosomes Is Monitored by the Mitotic Exit Network889
Cell-Cycle Arrest of Cells with Damaged DNA Depends on Tumor Suppressors891
20.8 Meiosis:A Special Type of Cell Division892
Key Features Distinguish Meiosis from Mitosis892
Repression of G1 Cyclins and a Meiosis-Specific Protein Kinase Promote Premeiotic S Phase895
Recombination and a Meiosis-Specific Cohesin Subunit Are Necessary for the Specialized Chromosome Segregation in Meiosis
热门推荐
- 2808814.html
- 3206666.html
- 3732719.html
- 3246186.html
- 2317168.html
- 2987529.html
- 2857211.html
- 2884110.html
- 3196142.html
- 2016247.html
- http://www.ickdjs.cc/book_2616836.html
- http://www.ickdjs.cc/book_2202143.html
- http://www.ickdjs.cc/book_1348715.html
- http://www.ickdjs.cc/book_1420698.html
- http://www.ickdjs.cc/book_1574981.html
- http://www.ickdjs.cc/book_3684487.html
- http://www.ickdjs.cc/book_3059163.html
- http://www.ickdjs.cc/book_3620745.html
- http://www.ickdjs.cc/book_706924.html
- http://www.ickdjs.cc/book_3441497.html